В химии многогранная скелетная теория электронной пары (PSEPT) обеспечивает подсчет электронов правила полезен для прогнозирования структуры кластеров , таких как боран и карборановых кластеры. Правила счета электронов были первоначально сформулированы Кеннетом Уэйдом [1] и в дальнейшем развиты Майклом Мингосом [2] и другими; их иногда называют правилами Уэйда или правилами Уэйда-Минго . [3] Правила основаны на молекулярной орбитальной обработке связи. [4] [5] [6][7] Эти правила были расширены и объединены в виде Jemmis MnO правил . [8] [9]
Прогнозирование структуры кластерных соединений [ править ]
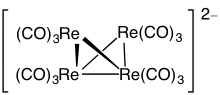
В зависимости от количества электронов на вершину используются разные правила (4 n , 5 n или 6 n ).
Правила 4 n достаточно точны для предсказания структур кластеров, имеющих около 4 электронов на вершину, как в случае многих боранов и карборанов . Для таких кластеров структуры основаны на дельтаэдрах , которые представляют собой многогранники, в которых каждая грань имеет треугольную форму. В 4 л кластеры классифицируются как closo- , nido- , arachno- или hypho- , на основе , представляют ли они полный ( closo- ) deltahedron , или deltahedron , что отсутствует один ( nido- ), два ( arachno- ) или три (дефо- ) вершины.
Однако гифокластеры относительно редки из-за того, что количество электронов достаточно велико, чтобы начать заполнять разрыхляющие орбитали и дестабилизировать структуру 4 n . Если количество электронов близко к 5 электронам на вершину, структура часто меняется на структуру, подчиняющуюся правилам 5n, которые основаны на трехсвязных многогранниках.
В качестве количества электронов при дальнейшем увеличении, структуры кластеров с помощью 5ны отсчетов электронов становятся нестабильными, поэтому 6 п правил могут быть реализованы. 6 n кластеров имеют структуры, основанные на кольцах.
Обработка молекулярных орбиталей может быть использована для рационализации связывания кластерных соединений типов 4 n , 5 n и 6 n .
4 n правил [ править ]
Следующие многогранники являются закрытыми многогранниками и являются основой для 4 n правил; у каждого из них треугольные грани. [10] Количество вершин в кластере определяет, на каком многограннике построена структура.
Количество вершин | Многогранник |
---|---|
4 | Тетраэдр |
5 | Тригональная бипирамида |
6 | Октаэдр |
7 | Пятиугольная бипирамида |
8 | D 2d (тригональный) додекаэдр ( курносый дисфеноид ) |
9 | Трехгранная тригональная призма |
10 | Двуглавая квадратная антипризма |
11 | Икосаэдр со сжатыми ребрами (октадекаэдр) |
12 | Икосаэдр (двуглавая пятиугольная антипризма) |
С помощью подсчета электронов можно найти предсказанную структуру. n - количество вершин в кластере. 4 n правил перечислены в следующей таблице.
Электронный счет | Имя | Прогнозируемая структура |
---|---|---|
4 п - 2 | Двуглавый Closo | n - 2-вершинный замкнутый многогранник с 2-мя закрытыми ( дополненными ) гранями |
4 п | блокированного клозо | n − 1 vertex closo polyhedron with 1 face capped |
4n + 2 | closo | closo polyhedron with n vertices |
4n + 4 | nido | n + 1 vertex closo polyhedron with 1 missing vertex |
4n + 6 | arachno | n + 2 vertex closo polyhedron with 2 missing vertices |
4n + 8 | hypho | n + 3 vertex closo polyhedron with 3 missing vertices |
4n + 10 | klado | n + 4 vertex closo polyhedron with 4 missing vertices |
10
When counting electrons for each cluster, the number of valence electrons is enumerated. For each transition metal present, 10 electrons are subtracted from the total electron count. For example, in Rh6(CO)16 the total number of electrons would be 6 × 9 + 16 × 2 − 6 × 10 = 86 – 60 = 26. Therefore, the cluster is a closo polyhedron because n = 6, with 4n + 2 = 26.
4
Other rules may be considered when predicting the structure of clusters:
- For clusters consisting mostly of transition metals, any main group elements present are often best counted as ligands or interstitial atoms, rather than vertices.
- Larger and more electropositive atoms tend to occupy vertices of high connectivity and smaller more electronegative atoms tend to occupy vertices of low connectivity.
- In the special case of boron hydride clusters, each boron atom connected to 3 or more vertices has one terminal hydride, while a boron atom connected to two other vertices has two terminal hydrogen atoms. If more hydrogen atoms are present, they are placed in open face positions to even out the coordination number of the vertices.
- For the special case of transition metal clusters, ligands are added to the metal centers to give the metals reasonable coordination numbers, and if any hydrogen atoms are present they are placed in bridging positions to even out the coordination numbers of the vertices.
In general, closo structures with n vertices are n-vertex polyhedra.
To predict the structure of a nido cluster, the closo cluster with n + 1 vertices is used as a starting point; if the cluster is composed of small atoms a high connectivity vertex is removed, while if the cluster is composed of large atoms a low connectivity vertex is removed.
To predict the structure of an arachno cluster, the closo polyhedron with n + 2 vertices is used as the starting point, and the n + 1 vertex nido complex is generated by following the rule above; a second vertex adjacent to the first is removed if the cluster is composed of mostly small atoms, a second vertex not adjacent to the first is removed if the cluster is composed mostly of large atoms.
Example: Pb2−
10
- Electron count: 10 × Pb + 2 (for the negative charge) = 10 × 4 + 2 = 42 electrons.
- Since n = 10, 4n + 2 = 42, so the cluster is a closo bicapped square antiprism.
Example: S2+
4
- Electron count: 4 × S – 2 (for the positive charge) = 4 × 6 – 2 = 22 electrons.
- Since n = 4, 4n + 6 = 22, so the cluster is arachno.
- Starting from an octahedron, a vertex of high connectivity is removed, and then a non-adjacent vertex is removed.
Example: Os6(CO)18
- Electron count: 6 × Os + 18 × CO – 60 (for 6 osmium atoms) = 6 × 8 + 18 × 2 – 60 = 24
- Since n = 6, 4n = 24, so the cluster is capped closo.
- Starting from a trigonal bipyramid, a face is capped. The carbonyls have been omitted for clarity.
5H4−
5, hydrogen atoms omitted
Example:[11] B
5H4−
5
- Electron count: 5 × B + 5 × H + 4 (for the negative charge) = 5 × 3 + 5 × 1 + 4 = 24
- Since n = 5, 4n + 4 = 24, so the cluster is nido.
- Starting from an octahedron, one of the vertices is removed.
The rules are useful in also predicting the structure of carboranes. Example: C2B7H13
- Electron count = 2 × C + 7 × B + 13 × H = 2 × 4 + 3 × 7 + 13 × 1 = 42
- Since n in this case is 9, 4n + 6 = 42, the cluster is arachno.
The bookkeeping for deltahedral clusters is sometimes carried out by counting skeletal electrons instead of the total number of electrons. The skeletal orbital (electron pair) and skeletal electron counts for the four types of deltahedral clusters are:
- n-vertex closo: n + 1 skeletal orbitals, 2n + 2 skeletal electrons
- n-vertex nido: n + 2 skeletal orbitals, 2n + 4 skeletal electrons
- n-vertex arachno: n + 3 skeletal orbitals, 2n + 6 skeletal electrons
- n-vertex hypho: n + 4 skeletal orbitals, 2n + 8 skeletal electrons
The skeletal electron counts are determined by summing the total of the following number of electrons:
- 2 from each BH unit
- 3 from each CH unit
- 1 from each additional hydrogen atom (over and above the ones on the BH and CH units)
- the anionic charge electrons
5n rules[edit]
As discussed previously, the 4n rule mainly deals with clusters with electron counts of 4n + k, in which approximately 4 electrons are on each vertex. As more electrons are added per vertex, the number of the electrons per vertex approaches 5. Rather than adopting structures based on deltahedra, the 5n-type clusters have structures based on a different series of polyhedra known as the 3-connected polyhedra, in which each vertex is connected to 3 other vertices. The 3-connected polyhedra are the duals of the deltahedra. The common types of 3-connected polyhedra are listed below.
Number of vertices | Type of 3-connected polyhedron |
---|---|
4 | Tetrahedron |
6 | Trigonal prism |
8 | Cube |
10 | Pentagonal prism |
12 | D2d pseudo-octahedron (dual of snub disphenoid) |
14 | Dual of triaugmented triangular prism (K5 associahedron) |
16 | Square truncated trapezohedron |
18 | Dual of edge-contracted icosahedron |
20 | Dodecahedron |
The 5n rules are as follows.
Total electron count | Predicted structure |
---|---|
5n | n-vertex 3-connected polyhedron |
5n + 1 | n – 1 vertex 3-connected polyhedron with one vertex inserted into an edge |
5n + 2 | n – 2 vertex 3-connected polyhedron with two vertices inserted into edges |
5n + k | n − k vertex 3-connected polyhedron with k vertices inserted into edges |
Example: P4
- Electron count: 4 × P = 4 × 5 = 20
- It is a 5n structure with n = 4, so it is tetrahedral
Example: P4S3
- Electron count 4 × P + 3 × S = 4 × 5 + 3 × 6 = 38
- It is a 5n + 3 structure with n = 7. Three vertices are inserted into edges
Example: P4O6
- Electron count 4 × P + 6 × O = 4 × 5 + 6 × 6 = 56
- It is a 5n + 6 structure with n = 10. Six vertices are inserted into edges
6n rules[edit]
As more electrons are added to a 5n cluster, the number of electrons per vertex approaches 6. Instead of adopting structures based on 4n or 5n rules, the clusters tend to have structures governed by the 6n rules, which are based on rings. The rules for the 6n structures are as follows.
Total electron count | Predicted structure |
---|---|
6n – k | n-membered ring with k⁄2 transannular bonds |
6n – 4 | n-membered ring with 2 transannular bonds |
6n – 2 | n-membered ring with 1 transannular bond |
6n | n-membered ring |
6n + 2 | n-membered chain (n-membered ring with 1 broken bond) |
Example: S8
- Electron count = 8 × S = 8 × 6 = 48 electrons.
- Since n = 8, 6n = 48, so the cluster is an 8-membered ring.
Hexane (C6H14)
- Electron count = 6 × C + 14 × H = 6 × 4 + 14 × 1 = 38
- Since n = 6, 6n = 36 and 6n + 2 = 38, so the cluster is a 6-membered chain.
Isolobal vertex units[edit]
Provided a vertex unit is isolobal with BH then it can, in principle at least, be substituted for a BH unit, even though BH and CH are not isoelectronic. The CH+ unit is isolobal, hence the rules are applicable to carboranes. This can be explained due to a frontier orbital treatment.[10] Additionally there are isolobal transition-metal units. For example, Fe(CO)3 provides 2 electrons. The derivation of this is briefly as follows:
- Fe has 8 valence electrons.
- Each carbonyl group is a net 2 electron donor after the internal σ- and π-bonding are taken into account making 14 electrons.
- 3 pairs are considered to be involved in Fe–CO σ-bonding and 3 pairs are involved in π-backbonding from Fe to CO reducing the 14 to 2.
Bonding in cluster compounds[edit]
- closo-B
6H2−
6
6H2−
6 showing the orbitals responsible for forming the cluster. Pictorial representations of the orbitals are shown; the MO sets of T and E symmetry will each have two or one additional pictorial representation, respectively, that are not shown here.
- The boron atoms lie on each vertex of the octahedron and are sp hybridized.[11] One sp-hybrid radiates away from the structure forming the bond with the hydrogen atom. The other sp-hybrid radiates into the center of the structure forming a large bonding molecular orbital at the center of the cluster. The remaining two unhybridized orbitals lie along the tangent of the sphere like structure creating more bonding and antibonding orbitals between the boron vertices.[8] The orbital diagram breaks down as follows:
- The 18 framework molecular orbitals, (MOs), derived from the 18 boron atomic orbitals are:
- 1 bonding MO at the center of the cluster and 5 antibonding MOs from the 6 sp-radial hybrid orbitals
- 6 bonding MOs and 6 antibonding MOs from the 12 tangential p-orbitals.
- The 18 framework molecular orbitals, (MOs), derived from the 18 boron atomic orbitals are:
- The total skeletal bonding orbitals is therefore 7, i.e. n + 1.
Transition metal clusters[edit]
Transition metal clusters use the d orbitals for bonding. Thus they have up to nine bonding orbitals, instead of only the four present in boron and main group clusters.[12][13]
Clusters with interstitial atoms[edit]
Owing their large radii, transition metals generally form clusters that are larger than main group elements. One consequence of their increased size, these clusters often contain atoms at their centers. A prominent example is [Fe6C(CO)16]2-. In such cases, the rules of electron counting assume that the interstitial atom contributes all valence electrons to cluster bonding. In this way, [Fe6C(CO)16]2- is equivalent to [Fe6(CO)16]6- or [Fe6(CO)18]2-.[14]
References[edit]
- ^ Wade, K. (1971). "The structural significance of the number of skeletal bonding electron-pairs in carboranes, the higher boranes and borane anions, and various transition-metal carbonyl cluster compounds". J. Chem. Soc. D. 1971: 792–793. doi:10.1039/C29710000792.
- ^ Mingos, D. M. P. (1972). "A General Theory for Cluster and Ring Compounds of the Main Group and Transition Elements". Nature Physical Science. 236: 99–102. Bibcode:1972NPhS..236...99M. doi:10.1038/physci236099a0.
- ^ Welch, Alan J. (2013). "The significance and impact of Wade's rules". Chem. Commun. 49: 3615–3616. doi:10.1039/C3CC00069A.
- ^ Wade, K. (1976). "Structural and Bonding Patterns in Cluster Chemistry". Adv. Inorg. Chem. Radiochem. 18: 1–66. doi:10.1016/S0065-2792(08)60027-8. CS1 maint: discouraged parameter (link)
- ^ Girolami, G. (Fall 2008). "Lecture notes distributed at the University of Illinois, Urbana-Champaign". Cite journal requires
|journal=
(help) These notes contained original material that served as the basis of the sections on the 4n, 5n, and 6n rules. - ^ Gilespie, R. J. (1979). "Nyholm Memorial Lectures". Chem. Soc. Rev. 8 (3): 315–352. doi:10.1039/CS9790800315.
- ^ Mingos, D. M. P. (1984). "Polyhedral Skeletal Electron Pair Approach". Acc. Chem. Res. 17 (9): 311–319. doi:10.1021/ar00105a003. CS1 maint: discouraged parameter (link)
- ^ a b Jemmis, Eluvathingal D.; Balakrishnarajan, Musiri M.; Pancharatna, Pattath D. (2001). "A Unifying Electron-counting rule for Macropolyhedral Boranes, Metallaboranes, and Metallocenes". J. Am. Chem. Soc. 123 (18): 4313–4323. doi:10.1021/ja003233z. PMID 11457198.
- ^ Jemmis, Eluvathingal D.; Balakrishnarajan, Musiri M.; Pancharatna, Pattath D. (2002). "Electronic Requirements for Macropolyhedral Boranes". Chem. Rev. 102 (1): 93–144. doi:10.1021/cr990356x. PMID 11782130.
- ^ a b Cotton, F. Albert; Wilkinson, Geoffrey; Murillo, Carlos A.; Bochmann, Manfred (1999), Advanced Inorganic Chemistry (6th ed.), New York: Wiley-Interscience, ISBN 0-471-19957-5
- ^ a b Cotton, Albert (1990). Chemical Applications of Group Theory. John Wiley & Sons. pp. 205–251. ISBN 0-471-51094-7.
- ^ King, R. B.; Rouvray, D. H. (1977). "Chemical Applications of Group Theory and Topology.7. A Graph-Theoretical Interpretation of the Bonding Topology in Polyhedral Boranes, Carboranes, and Metal Clusters". J. Am. Chem. Soc. 99 (24): 7834–7840. doi:10.1021/ja00466a014.
- ^ Kostikova, G. P.; Korolkov, D. V. (1985). "Electronic Structure of Transition Metal Cluster Complexes with Weak- and Strong-field Ligands". Russ. Chem. Rev. 54 (4): 591–619. Bibcode:1985RuCRv..54..344K. doi:10.1070/RC1985v054n04ABEH003040.
- ^ Fehlner, Thomas P. (2006). "Cluster Compounds: Inorganometallic Compounds Containing Transition Metal & Main Group Elements". Encyclopedia of Inorganic Chemistry. doi:10.1002/0470862106.ia097. ISBN 0470860782.
General references[edit]
- Greenwood, Norman N.; Earnshaw, Alan (1997). Chemistry of the Elements (2nd ed.). Butterworth-Heinemann. ISBN 978-0-08-037941-8.
- Cotton, F. Albert; Wilkinson, Geoffrey; Murillo, Carlos A.; Bochmann, Manfred (1999), Advanced Inorganic Chemistry (6th ed.), New York: Wiley-Interscience, ISBN 0-471-19957-5